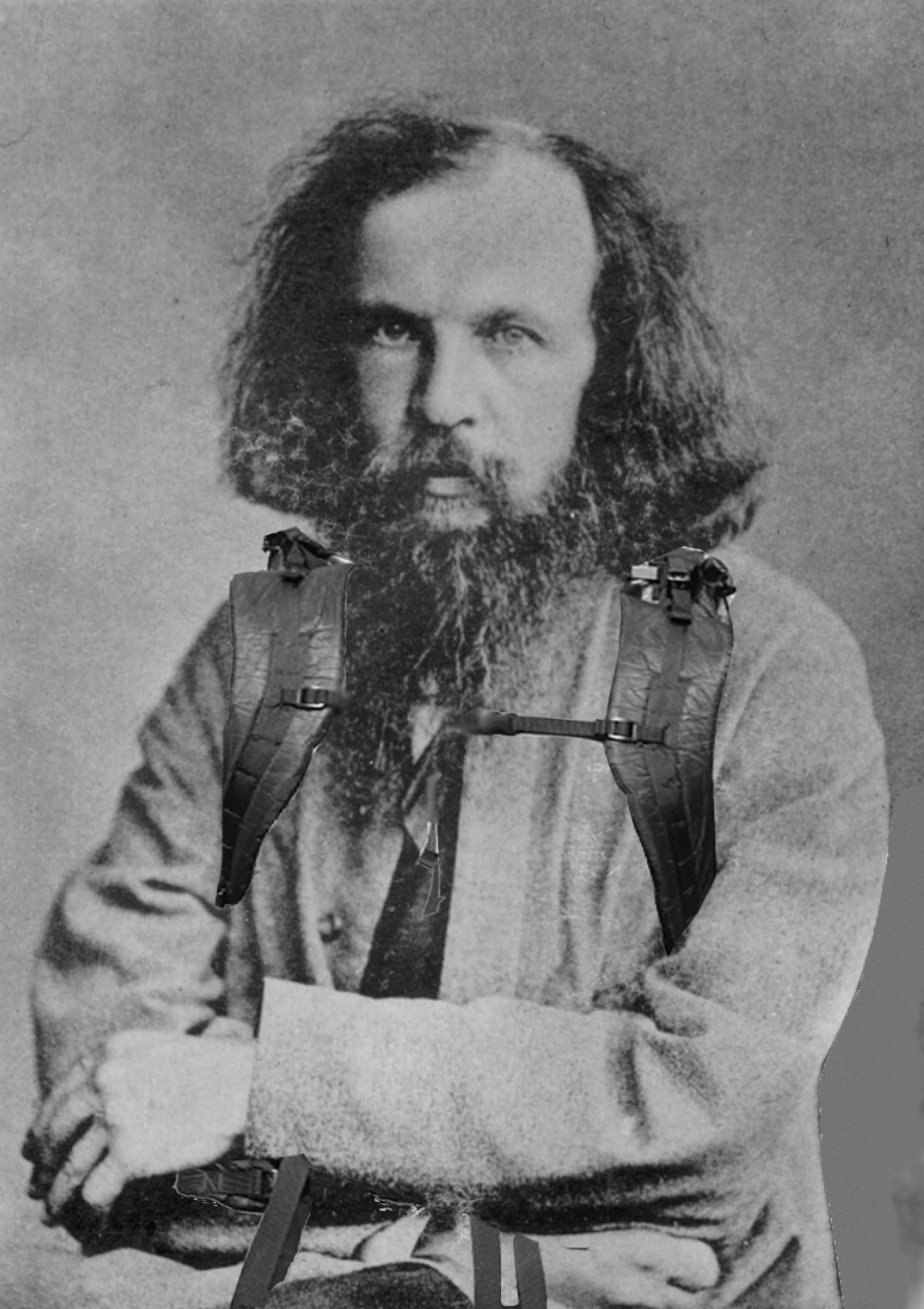
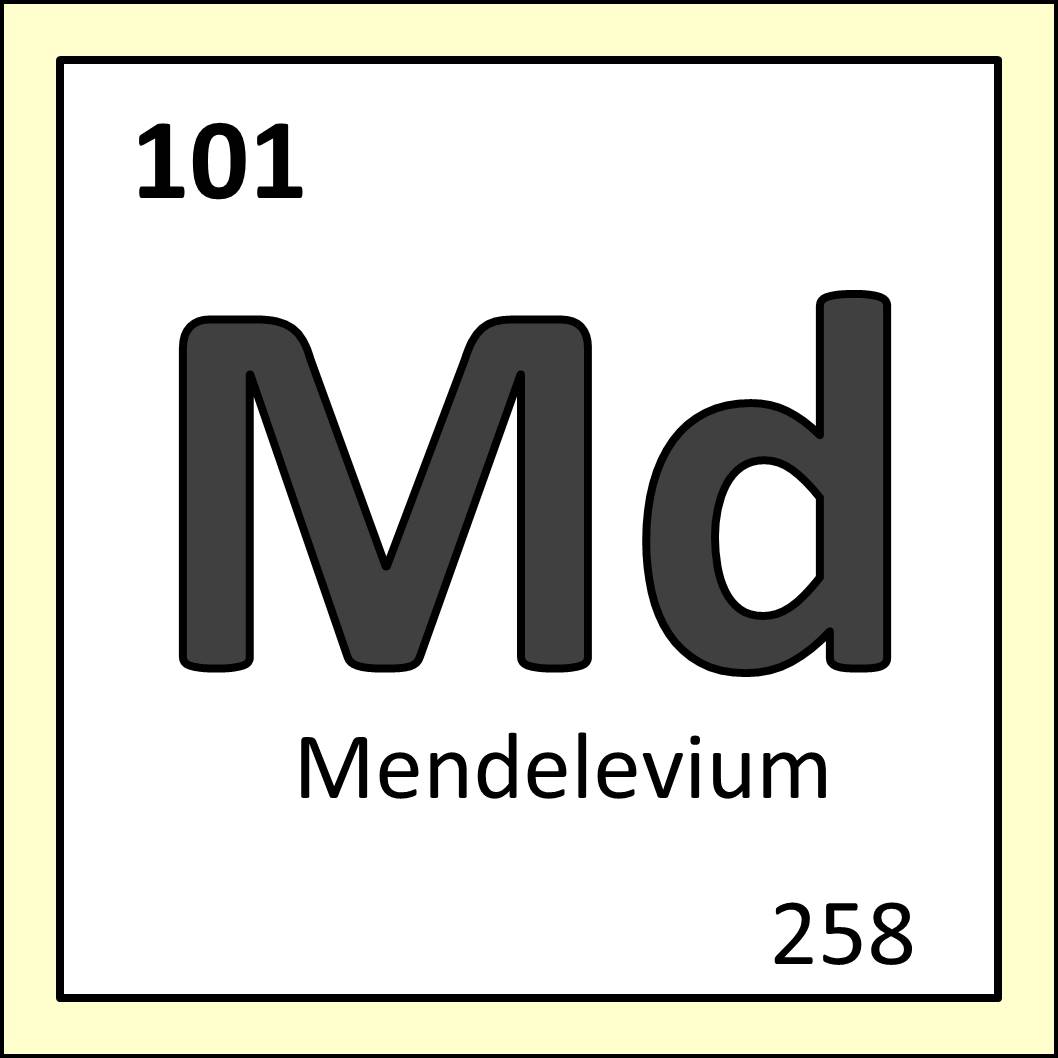
Left: Dmitri Mendeleev, From Wikimedia Commons. Right: Mendelevium as it appears on the periodic table.
Another day, another incredibly radioactive element: Mendelevium! A much more modern one, you can usually tell because there is very clearly an eminent scientist’s name in it. But more on that later…
Mendelevium is a synthetic element, which means that it does not appear naturally (on Earth at least) but instead must be made in a lab. It is also in the actinide series, a cohort of elements similar to actinium often depicted (along with the lanthanides) at the bottom of the periodic table. They appear here because even though they should be slotted between radium and rutherfordium, this would make the periodic table too long to read. The reason why they should sit there? Well for that we need to talk about electron shells.
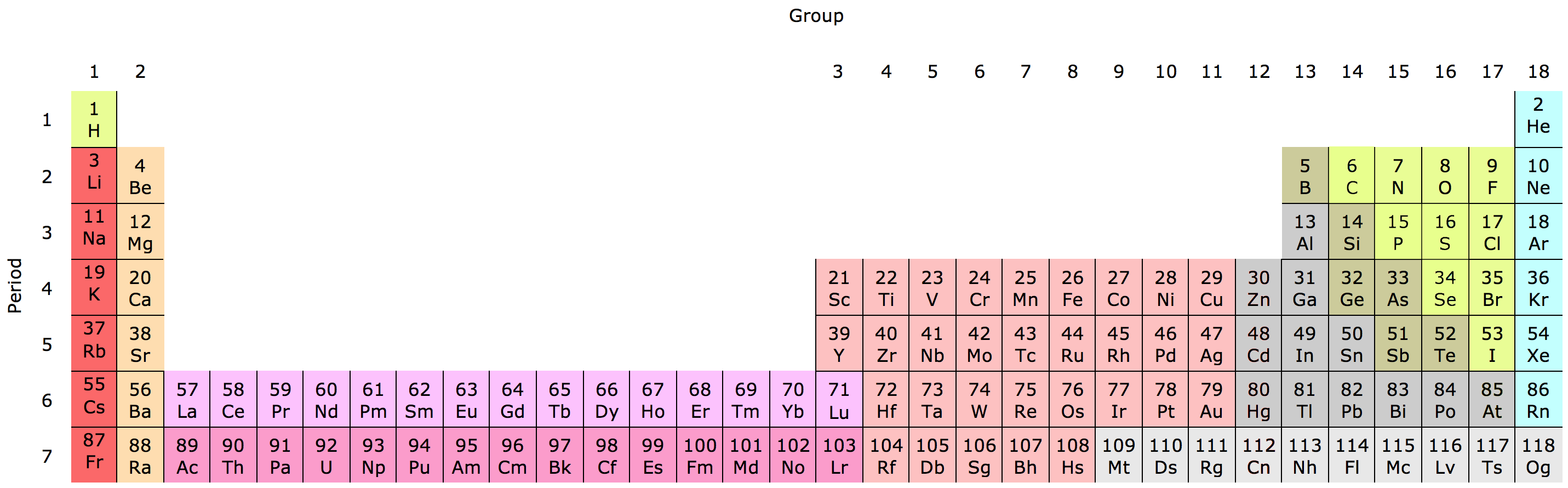
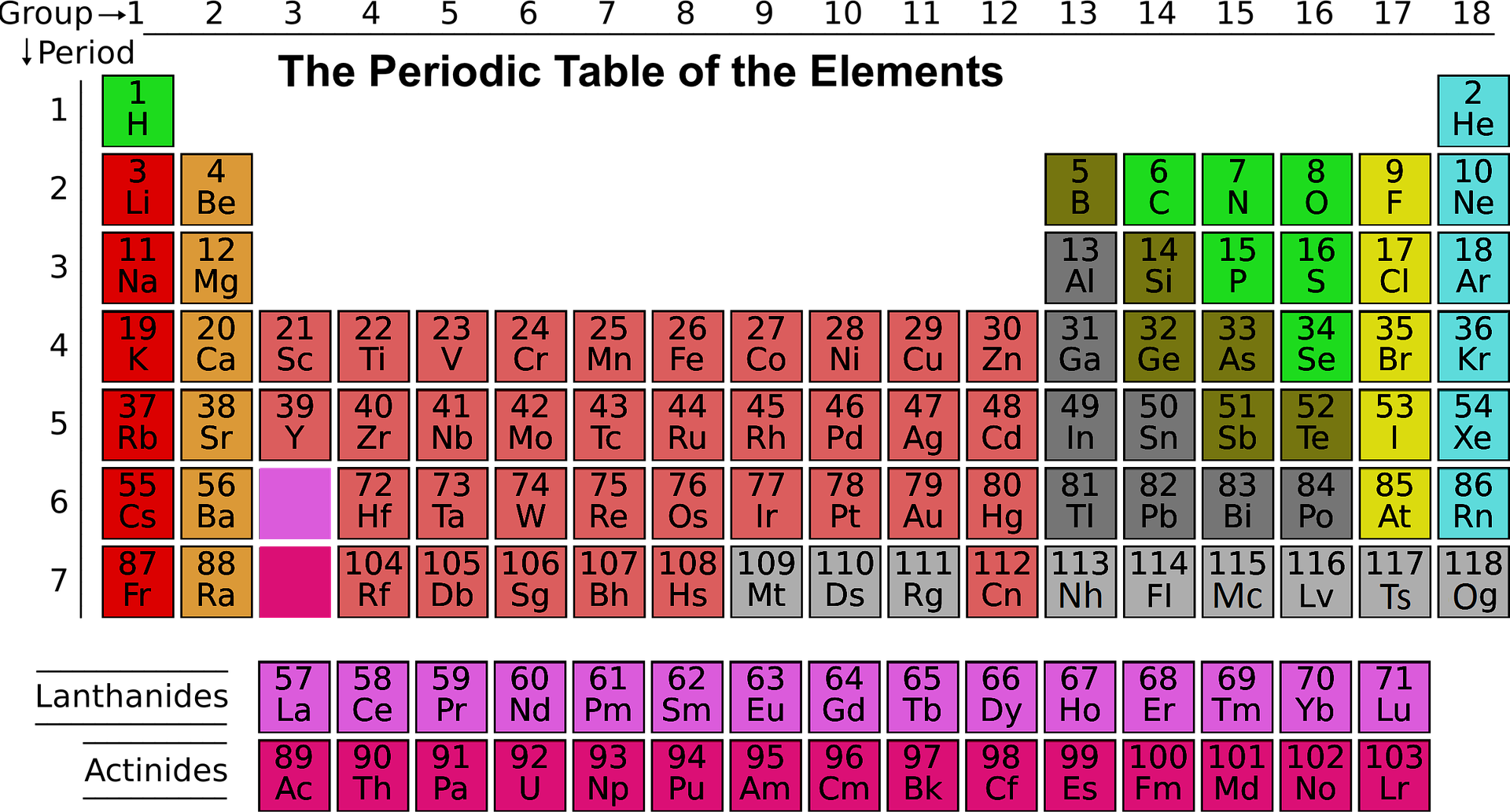
If the periodic table was arranged fully (top), the lanthanides and actinides would make it stretch out super long… In the modern periodic table (lower), the lanthanides and actinides are often plopped at the bottom. From Wikimedia Commons and Pixabay respectively.
An electron shell is an orbit around an atom’s nucleus that a set number of electrons can do. There is a limited number of electrons allowed in each shell, and therefore if another electron is added to an atom with a full shell, a new shell starts slightly further out to contain the new electron. This is why in some diagrams of an element’s atom there are those concentric circles with electrons on them; those are the shells that the electrons of that atom possess.
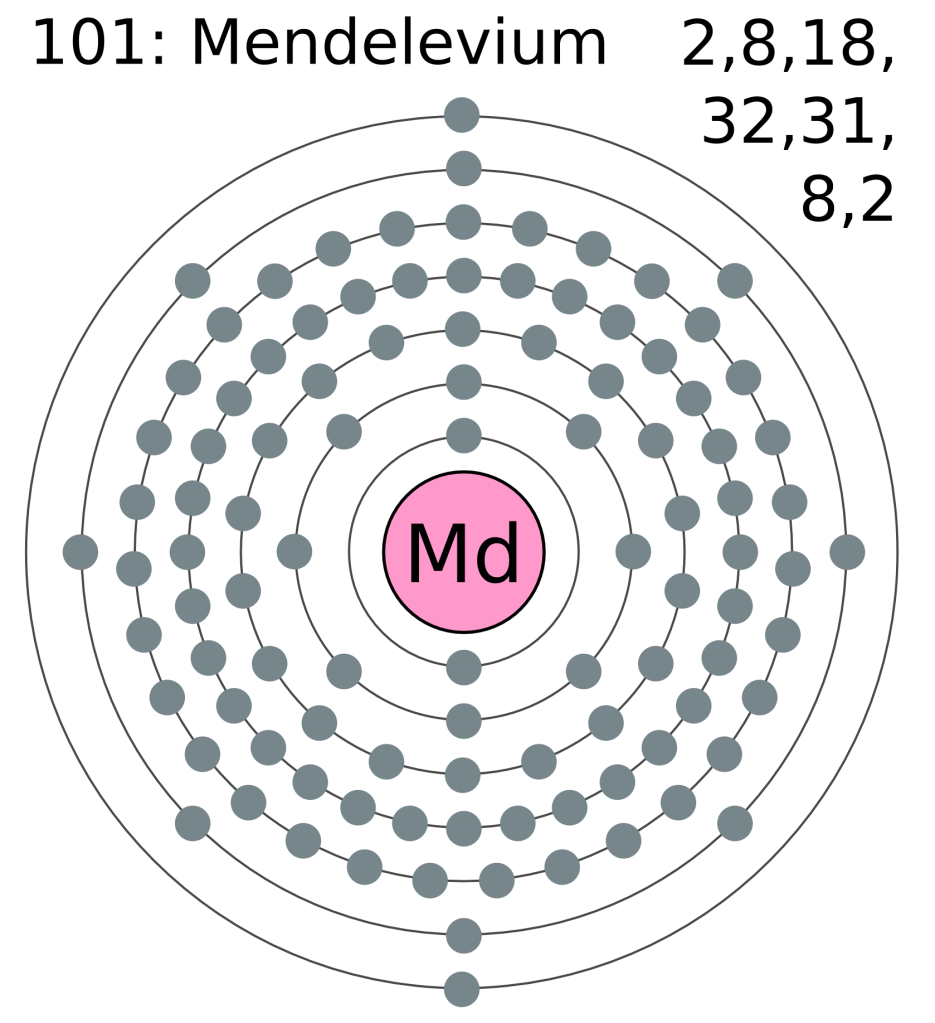
You may have noticed that some of these electron shells can hold more electrons than others. This is because each of these shells consist of a number of subshells. And it’s these subshells that we’re particularly interested in, as these are what gives the modern periodic table its structure. There are different types of subshell, and the further out the orbitals get, the more types of subshell they contain (hence why they mostly get bigger). I have coloured the subshells of mendelevium as best I can below:
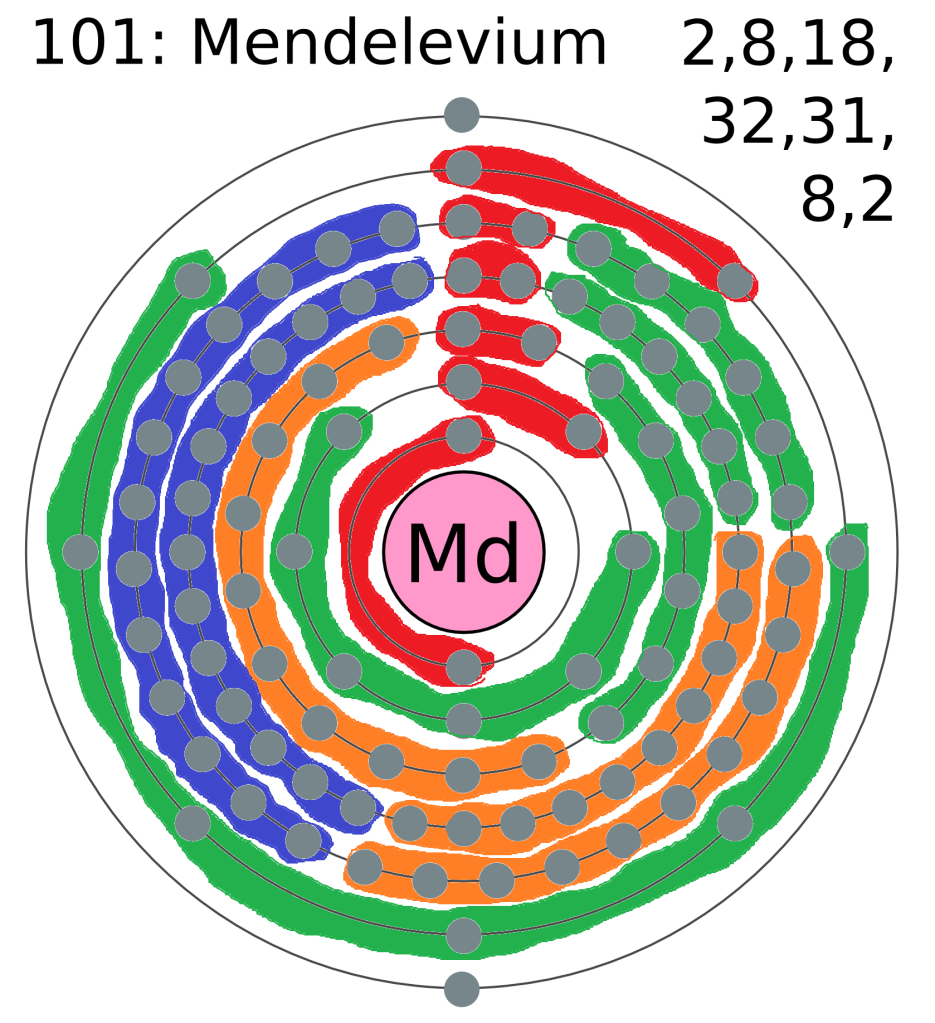
The first subshell is called s, and contains only one pair of electrons (also known as an orbital). The second subshell is called p, and contains 3 orbitals (6 electrons). The third is called d and contains 5 orbitals, and the fourth is called f and (usually) contains 7 orbitals. Looking at the diagram you will have noticed that the first shell only contains s, then with subsequent shells the next subshell is included, apart from outer two shells which are not technically full yet (because atoms couldn’t just be easy could they?). This is where the periodic table comes in, as the width of the different blocks (including our annoying lanthanides and actinides) actually fit the number of electrons that go into one of the subshells (as shown below). An annoying thing (which some of you beady eyed readers may have noticed) is that the d and f blocks appear to be in the row below where they should be. This is because of how the electron shells fill up which aren’t quite in the order of subshells (which is why the outer shells of mendelevium are weird). But the rule of thumb is that as you read the elements left to right across the periodic table, another electron is added to the shell. Which means that in the smaller atoms which don’t have d or f subshells, the next element jumps across the table from the s to the p block. So that’s the reason why the periodic table has that strange shape, and why the lanthanides and actinides should sit between the s and d blocks. Phew, thanks for sticking with me.
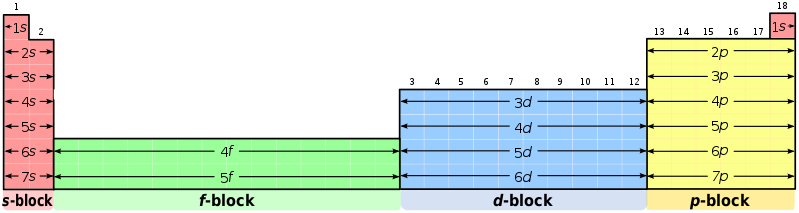
After all that subatomic chemistry, let’s talk about the discovery of mendelevium and the scientist it’s named after. As I said before, mendelevium doesn’t occur naturally and therefore rather than “discovered”, I guess it was “made” in 1955 by Stanley Thompson and his team at the University of California. They did this by bombarding einsteinium (yes that is a real element, just you wait until tomorrow) with alpha radiation- two protons and two neutrons stuck together, which increased the size of the einsteinium nuclei by two to make mendelevium. This new element was then named mendelevium after Dmitri Mendeleev, the creator of the periodic table back in the 19th century. As a small aside fact, mendelevium originally had the symbol Mv before it was changed to Md in 1957.
So there’s Mendelevium! Another radioactive element in the bag, but don’t worry; there are plenty more radioactive elements and plenty more elements left that are named after scientists. Watch this space…